
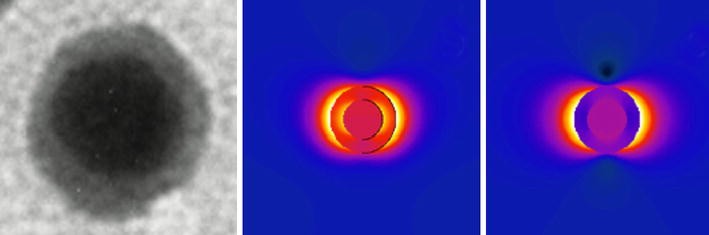
The figure is a presentation of a part of the heterogeneous antenna in the yz plane. For small distances, the symmetrically modes (bonding) and asymmetrically modes (anti-bonding) are governing the interaction modes. The plasmon mode shift can be explained by the strong interaction of the lower energy level with the highest ones in the energy level diagram presented in Figure 2a with red dashed lines. However, the resonant plasmon modes of LSPR in the NIR regions P.M.4 and P.M.5 blue shifts of 29.8 nm and 24.4 nm, respectively, as the interparticle distance increases. As the interparticle distance increases from 15 nm to 25 nm, the resonant plasmon modes of LSPR in the visible regions P.M.1, P.M.2 and P.M.3 redshifts of 50.0 nm, 4.0 nm and 18.3 nm, respectively. Figure 1f summarizes the plasmon resonance band shift as we modify the interparticle distance. By varying the interparticle distance in the zy plane from 15 nm to 25 nm, we noticed different variations and shifts of the LSPR plasmon modes. The two other plasmon bands denoted by plasmon mode 4 (P.M.4) and plasmon mode 5 (P.M.5) are located in the near-infrared regions at 822.1 nm and 927.0 nm, respectively, for d int = 15 nm. We then compared the effect of the surrounding medium covered by different chemical nature of solvents by studying the tuning sensitivity ( Δ λ max/ Δ n) that is defined as the change of the plasmon resonance peak position ( Δ λ max), expressed in nm, as a function of refractive index change ( Δ n), expressed in Refractive Index Unit (RIU). We compared pure gold and silver and heterogeneous nanostructure of core-shell nanospheres that decorate the Si wire nanoantenna. This was followed by a quantitative description of the significance of using shell nanolayer on pure silver. We studied the quantitative enhancement factor and plasmon modes when varying the interparticle distance between the surfaces of the gold shell in the interparticle junction in the z axis by keeping the distance between the surfaces of the gold shell in xy axis constant to 1 nm. We start our numerical simulation by optimization of the configuration of silicon nanoantenna decorated by Ag/Au core-shell nanospheres. In addition, it is necessary to optimize the geometric configuration of the nanoparticles in order to enhance their electric amplification factor (EF), thus making the materials useful in several applications such as surface enhanced Raman spectroscopy (SERS) and photocatalysis. Consequently, it is paramount to study more configurations of plasmonic amplifiers that are characterized by lower cost as well as chemically environmentally friendly. Besides, the amplification on the adjacent surface of NPs and catalytic material may be increased, leading to a hot-carrier generation. By coupling a catalytic material as a protective self-assembled monolayer to the nanoplasmonic NPs, the efficiency of plasmonic NPs is improved by tuning the plasmon resonance higher/lower wavelength therefore expanding the absorption response frequency of NPs. Unfortunately, some have limited surface chemistry such as Ag that degrades due to unstable chemical composition, high cost and are deficient optical absorbers as catalysts. Pure metallic NPs are the most used nanomaterials as plasmonic amplifiers due to the high rate of reproducibility and durability of, i.e., Au. The enhancement intensity around NPs is strongly dependent on the nanoparticles chemical composition as well as their geometric features (radius and orientation), the latter stay the most interesting factors to be considered firstly in any nanoplasmonic amplifiers. Therefore, they are known as excellent UV-Vis-NIR emitters. Another feature of nanoplasmonic NPs is the exited wide range of wavelengths, extending from the ultraviolet (UV)-visible (V) to near infrared (NIR).
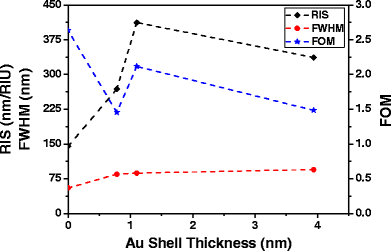
As a result, the concentrated light generates a strong intensity of the electromagnetic field (enhanced field (EF)) of several orders of magnitude achieved either on the apex of NPs or by approaching two (dimer), three (trimer), four (quadrimer) or multiple NPs. While a part of this emitted light is scattered to the far field, the other part is concentrated in the nanoscale vicinity of the metal surface of NPs leading to nanoplasmonic NPs. Due to light excitation of the NPs, electronic oscillation is induced, and the NP behaves as an electromagnetic dipole re-emitting light coherently at the same frequency. LSPR are the oscillations of electron clouds around NPs.
